What is Characteristic Impedance?
Characteristic impedance, denoted as Z0, is a fundamental property of a transmission line that describes the ratio of voltage to current for a wave propagating along the line. It is determined by the geometric and material properties of the transmission line, such as conductor size, spacing, and dielectric material. Characteristic impedance is crucial in signal integrity analysis, as it affects signal reflection, transmission, and matching in high-speed digital and RF systems.
The characteristic impedance of a transmission line is given by the following equation:
Z0 = √(L/C)
Where:
– L is the inductance per unit length of the transmission line
– C is the capacitance per unit length of the transmission line
In an ideal lossless transmission line, the characteristic impedance is purely real and frequency-independent. However, in real-world scenarios, transmission lines have finite resistance and conductance, resulting in a complex and frequency-dependent characteristic impedance.
Types of Characteristic Impedance
There are two main types of characteristic impedance:
-
Real Characteristic Impedance: In lossless or low-loss transmission lines, the characteristic impedance is purely real and frequency-independent. This is the case for ideal transmission lines or lines with negligible resistance and conductance. Examples include air-dielectric coaxial cables and low-loss microstrip lines.
-
Complex Characteristic Impedance: In transmission lines with significant losses, the characteristic impedance becomes complex and frequency-dependent. The real part represents the resistive component, while the imaginary part represents the reactive component. The complex characteristic impedance can be expressed as:
Z0 = R0 + jX0
Where:
– R0 is the real part of the characteristic impedance
– X0 is the imaginary part of the characteristic impedance
Complex characteristic impedance is more common in high-frequency applications, such as RF and microwave systems, where conductor and dielectric losses become significant.
Factors Affecting Characteristic Impedance
Several factors influence the characteristic impedance of a transmission line:
-
Conductor Geometry: The cross-sectional shape and dimensions of the conductors, such as wire diameter, trace width, and thickness, affect the characteristic impedance. In general, larger conductor cross-sections result in lower characteristic impedance.
-
Dielectric Material: The dielectric material surrounding the conductors plays a crucial role in determining the characteristic impedance. The dielectric constant (εr) and loss tangent (tan δ) of the material influence the capacitance and conductance of the transmission line. Higher dielectric constants lead to lower characteristic impedance.
-
Conductor Spacing: The spacing between the conductors, such as the separation between the signal and ground planes in a microstrip line or the spacing between the center conductor and shield in a coaxial cable, affects the characteristic impedance. Larger spacing results in higher characteristic impedance.
-
Frequency: In real transmission lines with losses, the characteristic impedance varies with frequency. At higher frequencies, the skin effect and dielectric losses become more pronounced, leading to changes in the characteristic impedance.
-
Line Length: The length of the transmission line does not directly affect the characteristic impedance, but it determines the electrical length and the impact of losses on signal propagation. Longer lines experience greater attenuation and dispersion, which can affect the apparent characteristic impedance seen by the signal.
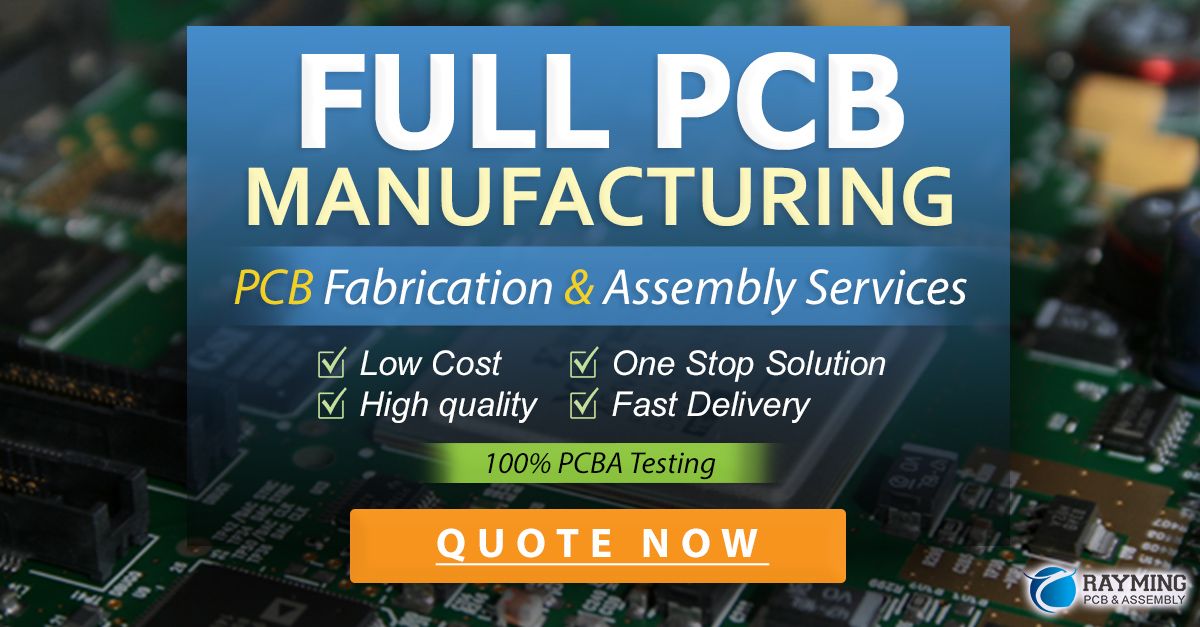
Common Characteristic Impedance Values
Different applications and transmission line types have standardized characteristic impedance values to ensure compatibility and optimal performance. Some common characteristic impedance values include:
Transmission Line Type | Characteristic Impedance (Ω) |
---|---|
Coaxial Cable (RG-6) | 75 |
Coaxial Cable (RG-58) | 50 |
Microstrip Line | 50, 75, 100 |
Stripline | 50, 75, 100 |
Twisted Pair (Ethernet) | 100 |
Waveguide (Rectangular) | Variable (based on dimensions) |
These values are chosen based on factors such as signal integrity requirements, power handling capacity, and compatibility with existing equipment and standards.
Importance of Matching Characteristic Impedance
Matching the characteristic impedance of a transmission line to the source and load impedances is essential for several reasons:
-
Reflection Reduction: When a signal encounters an impedance mismatch, a portion of the signal is reflected back towards the source. These reflections can cause signal distortion, ringing, and standing waves, degrading signal integrity. By matching the characteristic impedance, reflections are minimized, ensuring efficient power transfer and maintaining signal fidelity.
-
Transmission Efficiency: Impedance matching maximizes the power transfer from the source to the load. When the source impedance, characteristic impedance, and load impedance are matched, the maximum amount of power is delivered to the load, minimizing power loss in the transmission line.
-
Bandwidth Optimization: Proper impedance matching helps maintain a consistent frequency response over a wide bandwidth. Mismatched impedances can lead to frequency-dependent reflections and attenuation, limiting the usable bandwidth of the system.
-
Crosstalk Reduction: In multi-conductor transmission lines, such as ribbon cables or parallel traces on a PCB, matched characteristic impedances help reduce crosstalk between adjacent signals. By maintaining a consistent impedance profile, the coupling between signals is minimized, improving signal isolation and reducing interference.
Techniques for Achieving Impedance Matching
Several techniques can be employed to achieve impedance matching in transmission lines:
-
Termination Resistors: Adding a termination resistor at the end of the transmission line, with a value equal to the characteristic impedance, absorbs reflections and prevents standing waves. This technique is commonly used in high-speed digital systems to maintain signal integrity.
-
Impedance Transformers: Impedance transformers, such as quarter-wave transformers or tapered lines, can be used to match dissimilar impedances. These structures gradually change the impedance along the line, providing a smooth transition between the source and load impedances.
-
Matching Networks: Lumped-element matching networks, consisting of inductors and capacitors, can be designed to transform the load impedance to match the characteristic impedance of the transmission line. These networks are often used in RF and microwave applications.
-
Stub Matching: Open or short-circuited stubs, connected in parallel or series with the transmission line, can be used to cancel out reactances and match impedances. Stub matching is commonly employed in microstrip and stripline circuits.
-
Dielectric and Conductor Adjustments: By adjusting the dielectric material properties or the conductor geometry, such as trace width or spacing, the characteristic impedance can be tuned to match the desired value. This technique is often used during the PCB design phase to achieve controlled impedance traces.
FAQ
1. What is the difference between characteristic impedance and input impedance?
Characteristic impedance (Z0) is a property of the transmission line itself, determined by its geometric and material properties. It describes the ratio of voltage to current for a wave propagating along the line. On the other hand, input impedance (Zin) is the impedance seen looking into the transmission line from the source. Input impedance depends on the characteristic impedance, load impedance, and the length of the line.
2. How does the dielectric constant affect characteristic impedance?
The dielectric constant (εr) of the material surrounding the conductors directly influences the characteristic impedance. A higher dielectric constant results in a lower characteristic impedance. This is because the dielectric constant affects the capacitance per unit length of the transmission line. As the dielectric constant increases, the capacitance increases, leading to a decrease in the characteristic impedance (Z0 = √(L/C)).
3. What is the significance of a 50Ω characteristic impedance?
A 50Ω characteristic impedance has become a standard value in many RF, microwave, and high-speed digital applications. It offers a good compromise between power handling capability, low loss, and ease of manufacturing. Many connectors, cables, and components are designed to have a 50Ω impedance to ensure compatibility and optimal performance in 50Ω systems.
4. How can I measure the characteristic impedance of a transmission line?
There are several methods to measure the characteristic impedance of a transmission line:
a. Time Domain Reflectometry (TDR): TDR involves sending a fast-rising pulse down the transmission line and measuring the reflected signal. By analyzing the amplitude and timing of the reflections, the characteristic impedance can be determined.
b. Vector Network Analyzer (VNA): A VNA can measure the S-parameters of a transmission line over a range of frequencies. From the S-parameters, the characteristic impedance can be calculated using appropriate equations.
c. Impedance Analyzer: An impedance analyzer can directly measure the impedance of a transmission line at specific frequencies. By measuring the impedance at the desired frequency, the characteristic impedance can be obtained.
5. What is the impact of conductor surface roughness on characteristic impedance?
Conductor surface roughness can affect the characteristic impedance of a transmission line, particularly at high frequencies. Surface roughness increases the effective surface area of the conductor, leading to higher conductor losses and a slight increase in the characteristic impedance. The impact becomes more significant as the frequency increases and the skin depth decreases. To minimize the effect of surface roughness, techniques such as smooth copper plating or the use of low-profile copper foils can be employed.
Conclusion
Characteristic impedance is a crucial parameter in the design and analysis of transmission lines. It determines the behavior of signals propagating along the line and affects signal integrity, power transfer efficiency, and impedance matching. Understanding the factors that influence characteristic impedance, such as conductor geometry, dielectric material, and frequency, is essential for optimizing the performance of high-speed digital and RF systems.
By matching the characteristic impedance of the transmission line to the source and load impedances, reflections can be minimized, ensuring efficient power transfer and maintaining signal fidelity. Various techniques, including termination resistors, impedance transformers, matching networks, and stub matching, can be employed to achieve impedance matching.
Designers must carefully consider the characteristic impedance requirements of their applications and choose appropriate transmission line structures, materials, and dimensions to meet those requirements. Proper impedance control and matching are critical for the successful implementation of high-speed and high-frequency systems.
Leave a Reply