What is the Piezoelectric Effect?
The piezoelectric effect is the ability of certain crystalline materials to generate an electric charge in response to applied mechanical stress. The word piezoelectric comes from the Greek words “piezein”, which means to squeeze or press, and “electron” or amber, an ancient source of electric charge.
When piezoelectric material is placed under mechanical stress, a shifting of the positive and negative charge centers in the material takes place, which then results in an external electrical field. When reversed, an outer electrical field either stretches or compresses the piezoelectric material.
The piezoelectric effect is reversible, meaning:
- Applying mechanical stress/pressure generates an electrical charge (direct piezoelectric effect)
- Applying an electrical field creates mechanical strain/deformation (reverse piezoelectric effect)
Some common piezoelectric materials include:
Material | Formula |
---|---|
Quartz | SiO2 |
Topaz | Al2SiO4(F,OH)2 |
Tourmaline | (Ca,K,Na,)(Al,Fe,Li,Mg,Mn)3(Al,Cr, Fe,V)6(BO3)3(Si,Al,B)6O18(OH,F)4 |
Barium Titanate | BaTiO3 |
Lead Zirconate Titanate (PZT) | Pb[ZrxTi1−x]O3 0 ≤ x ≤ 1 |
History and Discovery of Piezoelectricity
The piezoelectric effect was first discovered by French physicists Jacques and Pierre Curie in 1880. They found that certain crystals, when subjected to mechanical strain, became electrically polarized and the degree of polarization was proportional to the applied strain.
Conversely, these same crystals would deform when exposed to an electric field. Over the next few decades, piezoelectricity remained more of a scientific curiosity.
The first practical application of piezoelectricity was during World War I. In 1917, French physicist Paul Langevin developed an ultrasonic submarine detector using a transducer made of thin quartz crystals bonded between two steel plates. This was the first sonar-type device.
The success of Langevin’s invention opened up opportunities for piezoelectric materials in underwater sound and ultrasonic applications. Since then, the development of man-made piezoelectric ceramics has greatly expanded the applications of piezoelectricity to the vast array of products on the market today.
How Does Piezoelectricity Work?
Crystal Structure and Dipole Moments
To understand how piezoelectricity works, we need to look at the crystal structure of piezoelectric materials. Piezoelectric crystals have a unique crystalline structure that is asymmetric and lacks a center of symmetry.
The asymmetry of the crystal lattice causes the formation of electric dipoles, which are created by the separation of positive and negative charges within the crystal structure. In the absence of external forces, these electric dipoles are randomly oriented, resulting in a net zero dipole moment.
Piezoelectric Coefficients and Constitutive Equations
The piezoelectric properties of a material are described by a set of piezoelectric coefficients. These coefficients relate the mechanical and electrical variables in the material. The most commonly used piezoelectric coefficients are:
- d: Direct piezoelectric coefficient (C/N or m/V)
- g: Voltage coefficient (Vm/N or m2/C)
- e: Stress coefficient (C/m2)
- h: Strain coefficient (V/m)
The constitutive equations for piezoelectricity describe the relationships between the mechanical and electrical variables in the material. These equations are:
- D = d·T + ε·E
- S = s·T + d·E
Where:
– D: Electric displacement (C/m2)
– T: Mechanical stress (N/m2)
– E: Electric field (V/m)
– S: Mechanical strain
– s: Elastic compliance (m2/N)
– ε: Permittivity (F/m)
Direct Piezoelectric Effect
In the direct piezoelectric effect, applying a mechanical stress to the piezoelectric material causes a change in the electric polarization, resulting in a charge buildup on the surface of the material. This charge can be collected and used to generate an electric current or voltage.
The direct piezoelectric effect is described by the equation:
D = d·T
Where D is the electric displacement, d is the piezoelectric coefficient, and T is the applied mechanical stress.
Reverse Piezoelectric Effect
The reverse piezoelectric effect is the opposite of the direct effect. When an electric field is applied to a piezoelectric material, it causes the material to deform or strain. The amount of strain is proportional to the applied electric field.
The reverse piezoelectric effect is described by the equation:
S = d·E
Where S is the mechanical strain, d is the piezoelectric coefficient, and E is the applied electric field.
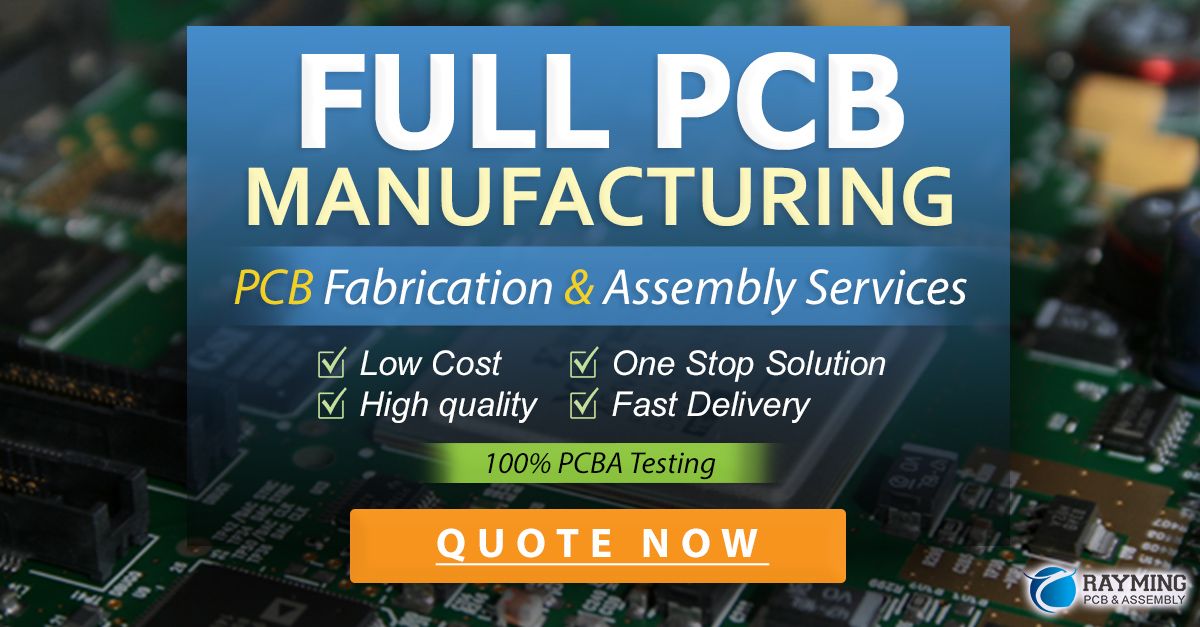
Piezoelectric Materials
Natural Piezoelectric Materials
Some naturally occurring materials exhibit piezoelectric properties. These include:
- Quartz (SiO2)
- Berlinite (AlPO4)
- Topaz (Al2SiO4(F,OH)2)
- Tourmaline-group minerals
- Cane sugar
- Rochelle salt (KNaC4H4O6·4H2O)
Among these, quartz is the most widely used natural piezoelectric material due to its excellent thermal stability and high mechanical quality factor.
Synthetic Piezoelectric Materials
With advancements in material science, several synthetic piezoelectric materials have been developed. These materials offer improved piezoelectric properties and can be tailored to specific applications. Some common synthetic piezoelectric materials include:
- Barium Titanate (BaTiO3)
- Lead Zirconate Titanate (PZT)
- Potassium Niobate (KNbO3)
- Lithium Niobate (LiNbO3)
- Lithium Tantalate (LiTaO3)
- Polyvinylidene Fluoride (PVDF)
PZT is one of the most widely used synthetic piezoelectric materials due to its strong piezoelectric effect and high Curie temperature.
Applications of Piezoelectricity
Piezoelectric materials have found applications in various fields due to their unique ability to convert mechanical energy into electrical energy and vice versa. Some of the main applications include:
Sensors and Transducers
Piezoelectric materials are used in a wide range of sensors and transducers, such as:
- Pressure sensors
- Force sensors
- Accelerometers
- Acoustic sensors (microphones, hydrophones)
- Ultrasonic transducers
These sensors and transducers are used in various industries, including automotive, aerospace, medical, and industrial automation.
Actuators and Motors
The reverse piezoelectric effect is utilized in actuators and motors. When an electric field is applied to a piezoelectric material, it deforms, causing precise and controlled motion. Some examples include:
- Piezoelectric motors
- Micro- and nanopositioning systems
- Fuel injectors
- Inkjet printer heads
- Camera lens focusing systems
Energy Harvesting
Piezoelectric materials can convert ambient mechanical energy (such as vibrations) into electrical energy. This property is used in energy harvesting applications, where the generated electricity can power small electronic devices or be stored for later use. Some examples include:
- Wearable electronics
- Wireless sensor networks
- Tire pressure monitoring systems
- Industrial machinery monitoring
Frequency Control and Timekeeping
The piezoelectric effect in quartz crystals is used in frequency control and timekeeping applications. Quartz crystal oscillators provide a stable and precise frequency reference for electronic devices. Some examples include:
- Quartz watches
- Radio and television broadcast stations
- GPS systems
- Computer clocks
Medical and Dental Applications
Piezoelectric materials are used in various medical and dental applications, such as:
- Ultrasonic imaging (sonography)
- Dental scaling devices
- Surgical instruments
- Bone growth stimulation
Advantages and Disadvantages of Piezoelectric Materials
Advantages
- High sensitivity and accuracy
- Fast response time
- Wide frequency range
- Low power consumption
- Compact size
- No moving parts (in some applications)
- Reversible effect (can be used as both sensors and actuators)
Disadvantages
- Limited strain and displacement
- Brittleness of some piezoelectric materials
- High voltage requirements for some applications
- Temperature sensitivity
- Aging and depolarization over time
- Relatively high cost compared to some alternative technologies
Future Developments and Research
As the demand for smart materials and systems continues to grow, piezoelectric materials are expected to play an increasingly important role in various applications. Some areas of ongoing research and development include:
- Nanoscale piezoelectric materials and devices
- Flexible and stretchable piezoelectric composites
- Bio-compatible piezoelectric materials for medical applications
- High-temperature piezoelectric materials
- Integration of piezoelectric materials with other smart materials (e.g., shape memory alloys)
- Optimization of energy harvesting systems using piezoelectric materials
Frequently Asked Questions (FAQ)
1. What is the difference between piezoelectricity and ferroelectricity?
Piezoelectricity is the ability of a material to generate an electric charge in response to applied mechanical stress, while ferroelectricity is a property of certain materials that have a spontaneous electric polarization that can be reversed by applying an external electric field. All ferroelectric materials are piezoelectric, but not all piezoelectric materials are ferroelectric.
2. Can piezoelectric materials generate electricity continuously?
Piezoelectric materials generate electricity only when they are subjected to a change in mechanical stress. They cannot generate electricity continuously unless there is a continuous change in the applied stress.
3. How long do piezoelectric materials last?
The lifetime of piezoelectric materials depends on various factors, such as the material composition, operating conditions, and the application. Some piezoelectric materials may suffer from aging and depolarization over time, which can affect their performance. However, with proper design and maintenance, piezoelectric devices can last for many years.
4. Are piezoelectric materials safe to use?
Most piezoelectric materials are safe to use in normal operating conditions. However, some piezoelectric materials, such as those containing lead (e.g., PZT), may pose health risks if not handled properly. It is important to follow proper safety guidelines and dispose of piezoelectric materials in accordance with local regulations.
5. How do I choose the right piezoelectric material for my application?
The choice of piezoelectric material depends on the specific requirements of the application, such as sensitivity, operating frequency, temperature range, and cost. Factors to consider include the piezoelectric coefficients, dielectric properties, mechanical properties, and environmental compatibility. It is advisable to consult with a piezoelectric material expert or supplier to select the most suitable material for your application.
Conclusion
Piezoelectricity is a fascinating phenomenon that has found numerous applications in various fields, from everyday devices to cutting-edge technologies. By understanding the principles behind piezoelectricity and the properties of piezoelectric materials, engineers and researchers can continue to develop innovative solutions to meet the evolving needs of our society.
As research in piezoelectric materials continues to advance, we can expect to see new and improved applications in areas such as energy harvesting, smart structures, and biomedical devices. The future of piezoelectricity is bright, and its potential to transform our world is limitless.
Leave a Reply